The Discovery of iPS Cells
In 2006, a Japanese researcher, Shinya Yamanaka, showed in mouse cells that four transcription factors are sufficient to restore differentiated body cells and the genetic information in their nucleus to the state of embryonic stem cells: Oct4, Sox2, Klf4, and c-Myc. This discovery surprised many scientists and changed our understanding of how cells work. Meanwhile, it has also been shown that with different combinations of defined factors, not only pluripotent cells from body cells arise, but cells of a germline can be transferred directly into another germline. For example, mesodermal fibroblasts can be directly transformed into ectodermal neurons. Researchers refer to this process as direct reprogramming. Reprogramming technologies are opening up new possibilities for the study and treatment of diseases today.
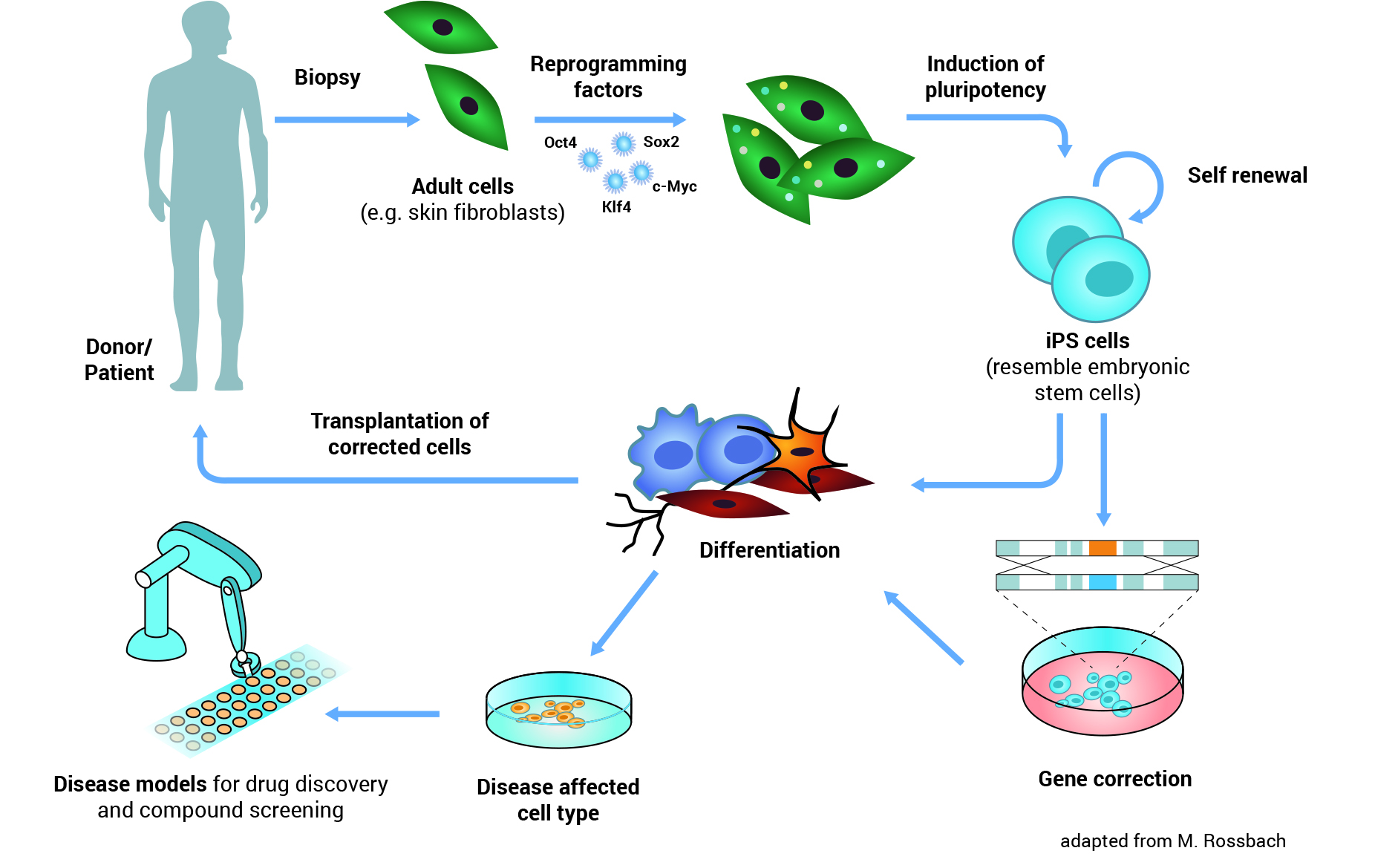
Illustration: iPS Cells - Origin and Application: iPS cells can be made from adult cells by introducing specific genes. The cells are thereby reprogrammed and pluripotent, similar to the embryonic stem cells. iPS cells can be differentiated to any cell type and then used for transplantation in cell replacement therapy. Similarly, cells affected by disease can be generated from iPS cells and used as a disease model in research or testing new drugs.
iPS and Embryonic Stem Cells
IPS cells and embryonic stem cells are very similar. They are self-renewing, meaning they can divide and produce copies of themselves indefinitely. Both types of stem cell can be used to derive nearly any kind of specialized cell under precisely controlled conditions in the laboratory. Both iPS cells and embryonic stem cells can help us understand how specialized cells develop from pluripotent cells. In the future, they might also provide an unlimited supply of replacement cells and tissues for many patients with currently untreatable diseases.
In contrast to embryonic stem cells, making iPS cells doesn’t depend on the use of cells from an early embryo. Are there any other differences? Current research indicates that some genes in iPS cells behave in a different way to those in embryonic stem cells. This is caused by incomplete reprogramming of the cells and/or genetic changes acquired by the iPS cells as they grow and multiply. Scientists are studying this in more detail to find out how such differences may affect the use of iPS cells in basic research and clinical applications. More research is also needed to understand just how reprogramming works inside the cell. So at the moment, most scientists believe we can’t replace ES cells with iPS cells in basic research.
iPS Cells and Disease
An important step in developing a therapy for a given disease is understanding exactly how the disease works: what exactly goes wrong in the body? To do this, researchers need to study the cells or tissues affected by the disease, but this is not always as simple as it sounds. For example, it’s almost impossible to obtain genuine brain cells from patients with Parkinson’s disease, especially in the early stages of the disease before the patient is aware of any symptoms. Reprogramming means scientists can now get access to large numbers of the particular type of neurons (brain cells) that are affected by Parkinson’s disease. Researchers first make iPS cells from, for example, skin biopsies from Parkinson’s patients. They then use these iPS cells to produce neurons in the laboratory. The neurons have the same genetic background (the same basic genetic make-up) as the patients’ own cells. Thus scientist can directly work with neurons affected by Parkinson’s disease in a dish. They can use these cells to learn more about what goes wrong inside the cells and why. Cellular ‘disease models’ like these can also be used to search for and test new drugs to treat or protect patients against the disease.
Future Applications of iPS-Zellen
Reprogramming holds great potential for new medical applications, such as cell replacement therapies. Since iPS cells can be made from a patient’s own skin, they could be used to grow specialized cells that exactly match the patient and would not be rejected by the immune system. If the patient has a genetic disease, the genetic problem could be corrected in their iPS cells in the laboratory, and these repaired iPS cells used to produce a patient-specific batch of healthy specialized cells for transplantation. But this benefit remains theoretical for now.
Until recently, making iPS cells involved permanent genetic changes inside the cell, which can cause tumours to form. Scientists have now developed methods for making iPS cells without this genetic modification. These new techniques are an important step towards making iPS-derived specialized cells that would be safe for use in patients. Further research is now needed to understand fully how reprogramming works and how iPS cells can be controlled and produced consistently enough to meet the high quality and safety requirements for use in the clinic.